I am interested in studying the production of heavy quarkonium states in heavy ion collisions. This is one of the studies that aims to obtain information
about possible formation of a Quark-Gluon Plasma in the laboratory.
These notes are meant to clarify what I mean in this two sentence summary 😉 I specially tried to make this page for graduate students, so you can get an idea on the physics questions that heavy ion research is trying to answer.
QCD & QGP
I do research in relativistic heavy ion collisions. I’m interested in studying QCD (Quantum Chromo-Dynamics), the theory of the strong interactions which fundamentally is at the heart of particles such as protons and neutrons. This is a theory whose main actors are quarks and gluons. There are many ways to study QCD and in particular in relativistic heavy ion collisions we are interested in trying to do it at a high energy density. The interesting aspect is that in our everyday world, we never see quarks and gluons, we only see the protons and neutrons formed by the complicated interaction of quarks and gluons at low energies. We say that the quarks and gluons are “confined”, so that loosely speaking one can think of protons and neutrons as a bag of quarks and gluons. The understanding of the nature of confinement in QCD is the subject of the 2004 Nobel Prize in Physics. At high energies, many theoretical calculations of QCD on large dedicated computers have indicated that a very different behaviour should emerge. The expectation is that at high energies, the quarks and gluons will be the main players, able to exist by themselves, and protons and neutrons will no longer exist.
The type of matter that should be formed at these high energy densities can be thought of another phase of QCD matter, and it has been dubbed the “Quak-Gluon Plasma”. This is only one of the interesting aspects of QCD, as the sketch illustrates.
QGP & Heavy Ions
Experimentally, we hope to reach the high-energy domain of QCD by doing collisions of heavy ions. For our purposes, heavy ions are the largest chunks of matter made out of protons and neutrons that we can conveniently obtain and manipulate in the laboratory (our technology is far away from being able to produce a collision of neutron stars at high energies!). For more than 25 years, physicists have been doing experiments colliding heavy ions to look for clues of behaviour indicative with formation of a QGP. The experiments have been gathering evidence not from doing a single measurement, but rather from studying how several different observables behave.
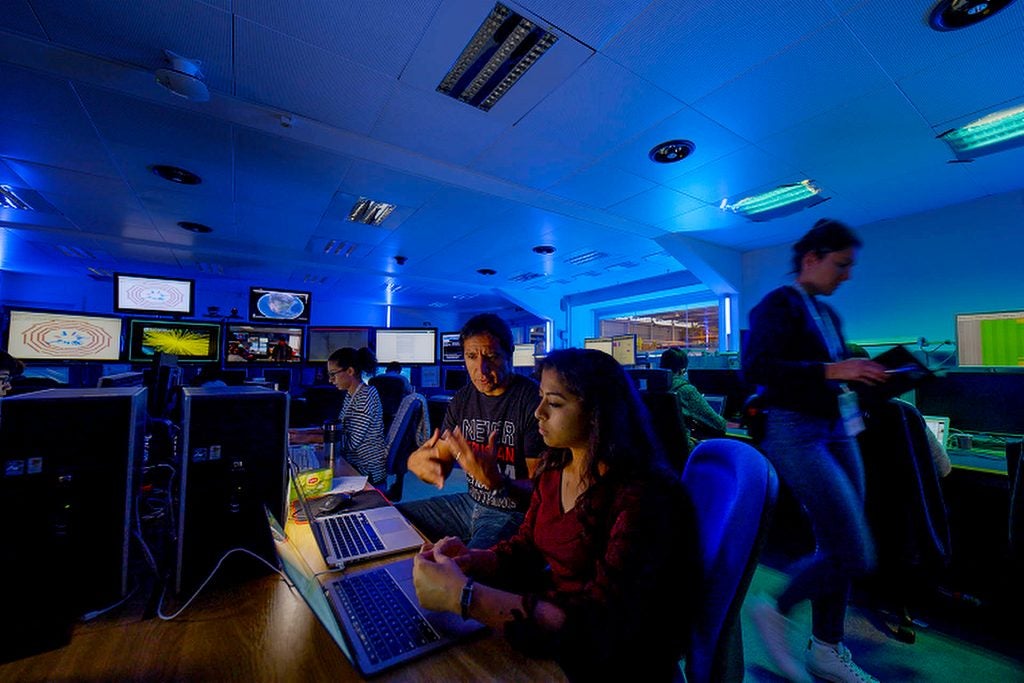
Analyzing heavy ion collisions at the CMS Center at CERN.
Heavy Quarkonium in heavy-ion collisions
Quarks are one of the building blocks of the Standard Model of particles and forces. They make up protons and neutrons, and also many other particles (that’s why they’re building blocks!). The Standard Model as we currently understand it, can be seen in a poster prepared by Berkeley Lab, shown here.
In particular, my current research interest is to measure heavy quark produc
tion in heavy ion collisions. The bound state of a quark-antiquark pair is commonly called “quarkonium”, in similar fashion as the bound state of an electron and its antiparticle, the positron, is called “positronium”. Specifically, heavy quark bound states are predicted to be significantly suppressed in the presence of a Quark-Gluon Plasma in an effect similar to Debye screening in an electrolyte: the color charge between the two heavy quarks is screened by the presence of the surrounding quarks and gluons. More recently, numerical calculations of QCD have refined this picture and provided a benchmark: different quarkonium states should dissolve at different temperatures. This is exciting news: a measurement of many of these states, with information as to which states have “melted” and which have not, should give us information about the temperature reached in the high energy heavy ion collisions. The temperatures produced in such collisions should exceed 200 MeV, or 2×1012 degrees K (the temperature of the sun’s core is 15×106degrees K, about a million degrees colder by comparison). That is how hot we need to make things in order to “melt” nuclear matter and produce quark matter!